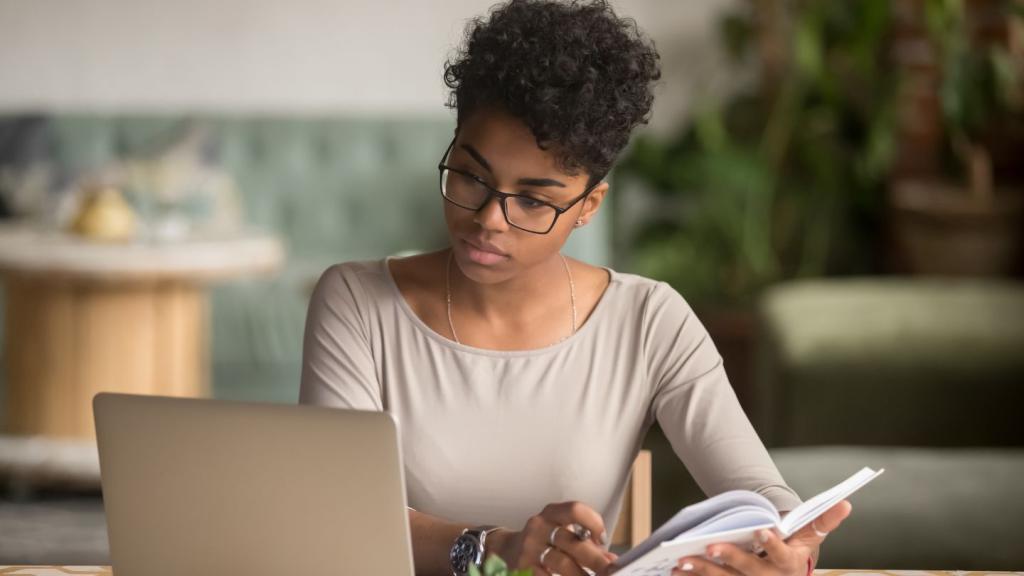
The immune system and primary immunodeficiency
Getting to know more about how the immune system works can help you better understand PI.
Getting to know more about how the immune system works can help you better understand PI.
The most common cells of the immune system can be categorized as lymphocytes (T cells, B cells, and NK cells), neutrophils, and monocytes/ macrophages. These are all types of white blood cells. The major proteins of the immune system are predominantly cytokines (a type of hormone responsible for communication between cells of the immune system), antibodies (immunoglobulins), and complement proteins.
B cells (sometimes called B-lymphocytes and often named on lab reports as CD19 or CD20 cells) are specialized cells of the immune system whose major function is to produce antibodies (also known as immunoglobulins or gamma-globulins). B cells develop in the bone marrow from stem cells. As part of their normal maturation in the bone marrow, B cells are trained or educated so that they do not produce antibodies to healthy tissues. When mature, B cells can be found in the bone marrow, lymph nodes, spleen, some areas of the intestine, and the bloodstream.
When B cells encounter foreign germs (antigens), they respond by maturing into another cell type called plasma cells. B cells can also mature into memory cells, which allows a rapid response if the same infection is encountered again. Plasma cells are the mature cells that actually produce the antibodies and are located in the spleen and lymph nodes throughout the body. Antibodies are highly specialized serum protein molecules that find their way into the bloodstream, tissues, respiratory secretions, intestinal secretions, and even tears. Collectively, plasma cells have the ability to produce antibodies against virtually all microbes in our environment. Each plasma cell, however, produces only one kind of antibody.
In fact, antibodies are actually specifically designed to recognize practically every germ that can cause infection. For every foreign antigen, there are antibody molecules specially designed to fit that antigen, like a lock and key. The variety of different antibody molecules found in a healthy immune system is vast. For example, there are specific antibody molecules that can recognize poliovirus, bacteria like diphtheria, the common cold virus, or the measles virus.
When antibody molecules recognize a microorganism as foreign, they physically attach to it and set off a complex chain of events involving other components of the immune system that work to eventually destroy the infection. Antibodies vary with respect to their specialized functions in the body. These variations are determined by the antibody’s chemical structure, which in turn determines the class of the antibody (or immunoglobulin).
Antibodies protect the body against infection in a number of different ways. For example, some microorganisms, such as viruses, must attach to body cells before they can cause an infection, but antibodies bound to the surface of a virus can interfere with the virus’s ability to attach to the host cell. In addition, antibodies attached to the surface of some microorganisms can cause the activation of a group of proteins called the complement system that can directly kill some bacteria. Antibody-coated bacteria are also much easier for neutrophils to ingest and kill than bacteria not coated with antibodies. All of these actions of antibodies prevent microorganisms from successfully invading body tissues and causing serious infections.
The long life of plasma cells enables us to retain immunity to viruses and bacteria that infected us many years ago. For example, once people have been fully immunized with live vaccine strains of the measles virus, they will almost never catch it because they retain the plasma cells and antibodies for many years, and these antibodies prevent infection.
T cells (sometimes called T lymphocytes and often named in lab reports as CD3 cells) are another type of immune cell. Some T cells directly attack cells infected with viruses, and others act as regulators of the immune system.
T cells develop from hematopoietic stem cells in the bone marrow but complete their development in the thymus. The thymus is a specialized organ of the immune system in the chest. Within the thymus, immature lymphocytes develop into mature T cells (the “T” stands for the thymus), and T cells with the potential to attack normal tissues are eliminated. The thymus is essential for this process, and T cells cannot develop if the fetus does not have a thymus. It is in the thymus that T cell receptor excision circles (TRECs) are made as a by-product of T cell maturation. (TRECs are measured in blood spots from newborn screening cards to identify infants with severe combined immunodeficiency (SCID) before they become sick with infections). Mature T cells leave the thymus as naïve T cells, ready to meet new antigens and populate other organs of the immune system, such as the spleen, lymph nodes, bone marrow, and blood, as memory T cells after these exposures to antigens.
Each T cell reacts with one specific antigen, just as each antibody molecule reacts with one specific antigen. In fact, T cells have molecules on their surfaces that are similar to antibodies. The variety of different T cells is also so extensive that the body has T cells that can react against virtually any antigen.
T cells have different abilities to recognize antigens and are varied in their function. There are killer or cytotoxic T cells (often denoted in lab reports as CD8 T cells), helper T cells (often denoted in lab reports as CD4 T cells), and regulatory T cells. Each has a different role to play in the immune system.
Killer, or cytotoxic T cells, perform the actual destruction of cells infected with viruses. Killer T cells protect the body from certain bacteria and viruses that have the ability to survive and even reproduce within the body’s own cells. In addition to fighting germs, killer T cells also recognize and respond to foreign tissues in the body, such as a transplanted kidney.
Helper T cells assist B cells to produce antibodies and assist killer T cells in their attack on foreign substances. The killer T cell must migrate to the site of infection and directly bind to its target to ensure its destruction.
When T cells are fighting infections, they grow and divide, making more T cells. Regulatory T cells suppress or turn off the T cells when an infection is controlled and are no longer needed. Without regulatory cells, the immune system would keep working even after an infection has been treated. Without regulatory T cells, there is the potential for the body to overreact to the infection. Regulatory T cells act as the thermostat of the lymphocyte system to keep it turned on just enough—not too much and not too little.
Natural killer (NK) cells are so named because they easily kill cells infected with viruses. They are said to be natural killer cells as they are always ready to fight and do not require the same thymic education that T cells require. NK cells are derived from the bone marrow and are present in relatively low numbers in the bloodstream and in tissues. They are important in defending against viruses and possibly preventing cancer as well.
NK cells kill virus-infected cells by injecting them with a killer potion of chemicals called cytotoxic granules. They are particularly important in the defense against herpes viruses. This family of viruses includes the traditional cold sore form of herpes (herpes simplex) as well as Epstein-Barr virus (the cause of infectious mononucleosis or mono) and the varicella virus (the cause of chickenpox and shingles).
Neutrophils or polymorphonuclear leukocytes (polys or PMNs) are the most numerous of all the types of white blood cells, making up about half or more of the total. They are also called granulocytes and appear on lab reports as part of a complete blood count (named in lab reports as CBC with differential). They are found in the bloodstream and can migrate into sites of infection within a matter of minutes. These cells, like the other cells in the immune system, develop from hematopoietic stem cells in the bone marrow.
Neutrophils increase in number in the bloodstream during infection and are in large part responsible for the elevated white blood cell count seen with some infections. They are the cells that leave the bloodstream and accumulate in the tissues during the first few hours of an infection and are responsible for the formation of pus. Their major role is to ingest bacteria or fungi and kill them. Their killing strategy relies on ingesting the infecting organisms in specialized pockets within the cell. Neutrophils contain toxic chemicals that fuse with the bacteria-containing pockets to kill the bacteria. Neutrophils have little role in the defense against viruses.
Monocytes are closely related to neutrophils and are found circulating in the bloodstream. They make up 5 to 10% of the white blood cells. They also line the walls of blood vessels in organs like the liver and spleen where they capture microorganisms in the blood as they pass by. When monocytes leave the bloodstream and enter the tissues, they change shape and size and become macrophages. Macrophages are essential for killing fungi and the class of bacteria to which tuberculosis belongs (mycobacteria). Like neutrophils, macrophages ingest microbes and deliver toxic chemicals directly to the foreign invader to kill it.
Macrophages live longer than neutrophils and are especially important for slow-growing or chronic infections. Macrophages can be influenced by T cells and often collaborate with T cells in killing microorganisms.
Immunodeficiencies are categorized as primary or secondary. Primary immunodeficiencies are 'primary' because an inherent defect in the immune system is the primary cause. Most are caused by genetic defects that may be inherited. Secondary immunodeficiencies are so called because they have been caused by other conditions including certain diseases or medications affecting the immune system.
The most common secondary immunodeficiencies are caused by aging, malnutrition, certain medications and some infections, such as human immunodeficiency virus or HIV. The most common medications associated with secondary immunodeficiencies are chemotherapy agents and immune suppressive medications, cancer, transplanted organ rejection, or autoimmune diseases. Other secondary immunodeficiencies include protein losses in the intestines or the kidneys. When proteins are lost, antibodies are also lost, leading to low immunoglobulins or low antibody levels. These conditions are important to recognize because, if the underlying cause can be corrected, the function of the immune system can be improved and/or restored. Regardless of the root cause, recognition of the secondary immunodeficiency and provision of immunologic support can be helpful. The types of support offered are comparable to what is used for primary immunodeficiencies.
Although forms of PI may differ from one another in many ways, they share one important feature. They all result from a defect in one or more of the elements or functions of the normal immune system, such as T cells, B cells, NK cells, neutrophils, monocytes, antibodies, cytokines, or the complement system. Most of them are inherited diseases and may run in families, such as X-linked agammaglobulinemia (XLA) or severe combined immune deficiency (SCID). Other primary immunodeficiencies, such as common variable immune deficiency (CVID) and selective IgA deficiency (SAD) are not always inherited in a clear-cut or predictable fashion. In these disorders, the cause is unknown, but it is believed that the interaction of genetic and environmental factors may play a role in causing them.
Because the most important function of the immune system is to protect against infection, people with PI have an increased susceptibility to infection. This may include too many infections, infections that are difficult to treat, unusually severe infections, or infections with unusual organisms. The infections may be located anywhere in the body. Common sites are the sinuses (sinusitis), the bronchi (bronchitis), the lung (pneumonia), and the intestinal tract (infectious diarrhea).
Another function of the immune system is to discriminate between the healthy tissue (“self”) and foreign material (“non-self”). Examples of foreign material can be microorganisms, pollen, or even a transplanted kidney from another individual. In some immunodeficiencies, the immune system is unable to discriminate between self and non-self. In these cases, in addition to an increased susceptibility to infection, people with PI may also have autoimmune diseases in which the immune system attacks their own cells or tissues as if these cells were foreign, or non-self.
There are also a few types of PI in which the ability to respond to an infection is largely intact, but the ability to regulate that response is abnormal. Examples of this are autoimmune lymphoproliferative syndrome (ALPS) and IPEX (X-linked syndrome of immunodeficiency, polyendocrinopathy, and enteropathy). These conditions are characterized by prominent autoimmunity where the body attacks itself.
PI can occur in individuals of any age. The original descriptions of these diseases were in children. As medical experience has grown, however, many adolescents and adults have been diagnosed with PI. This is partly due to the fact that some of the disorders, such as CVID and SAD, may have their initial clinical presentation in adult life.
Effective therapy exists for many forms of PI, and many people with these disorders can live relatively normal lives. PI was initially thought to be very rare. Recent research, however, has indicated that as a group they are more common than originally thought. It is estimated that as many as 1 in every 1,200-2,000 people may have some form of PI.
Getting diagnosed is the first step toward effective treatment of PI.
With proper medical care and treatment, many people with PI are able to live healthy and independent lives.
There are more than 450 primary immunodeficiencies with distinct definitions and symptoms.
Abnormalities in the immune system that lead to PI may also result in autoimmunity.
This page contains general medical and/or legal information that cannot be applied safely to any individual case. Medical and/or legal knowledge and practice can change rapidly. Therefore, this page should not be used as a substitute for professional medical and/or legal advice. Additionally, links to other resources and websites are shared for informational purposes only and should not be considered an endorsement by the Immune Deficiency Foundation.
Adapted from the IDF Patient & Family Handbook for Primary Immunodeficiency Diseases, Sixth Edition.
Copyright ©2019 by Immune Deficiency Foundation, USA
Receive news and helpful resources to your cell phone or inbox. You can change or cancel your subscription at any time.